Schiedam, November 16, 2023 – Hydrogen is one of modern times buzzwords. Every week, we hear of billions of dollars equivalent of projects being announced. So why does hydrogen attract so much attention? Is it only a short term trend or is it a trend that will last?
To start the discussion, let’s look at the following chart:
One can see on this chart, illustrating the mass energy density per fuel, that the fuels we are currently using have energy densities around 40MJ/kg in lower heating value. Hydrogen has three times the energy density per mass compared to most of the fuels we are using today.
But is it only recently that we discovered the energy density of hydrogen? Of course not, scientists have known about the energy density of hydrogen since the late 18th century.
Jules Verne in 1875 wrote in his book The Mysterious Island that: “Water is the coal of the future. Tomorrow’s energy is water that has been broken down by electric current. The elements of water thus decomposed, hydrogen and oxygen, will secure the earth’s energy supply for the unforeseeable time”. 150 years later, I feel like this could be the extract of a current scientific newspaper article.
Why did hydrogen not take-off at that moment? Or during the second industrial revolution?
Hydrogen poses a few technical challenges that proved to be unsolvable with the material science technologies of the time. For instance:
- Hydrogen is liquid at -253oC, which is the lowest liquefaction temperature of any chemical component known to humanity.
- Hydrogen has a minimum ignition energy of 0,02mJ, which is also the lowest among all components known. This means that it is extremely explosive. If you don’t believe me, just remember the Hindenbourg disaster of 1937, where Germany flew a Zepelin using Hydrogen.
Therefore, carbonated energy carriers, such as gasoline, diesel, kerosene, coal and later natural gas became the fuels of choice during the second industrial revolution.
What changed today, allowing hydrogen to make a come-back?
First, hydrogen has actually been used for the last 100 years, not as an energy carrier, but as an intermediate feedstock in chemical processes. For instance, half of the global hydrogen consumption currently comes from desulfurization of oil in refineries, and the other half is for ammonia production as a precursor for most nitrogen-based fertilizers. This allowed to gain experience about the molecule without having to deal with the drawback of transporting it, were it to be used as an energy carrier. As a matter of fact, large-scale alkaline electrolyzers have been in use for fertilizer production for over 100 years.
Then, a century of progress in material science, piping, mechanical and electrical science allow us to deal with the pressures, temperatures and fire safety requirements needed to handle and transport hydrogen safely.
Finally, climate change is forcing to scale back the use of fossil fuels that were mainly used as energy carriers in the past century, making the question of hydrogen once again a critical question.
What’s the future of hydrogen?
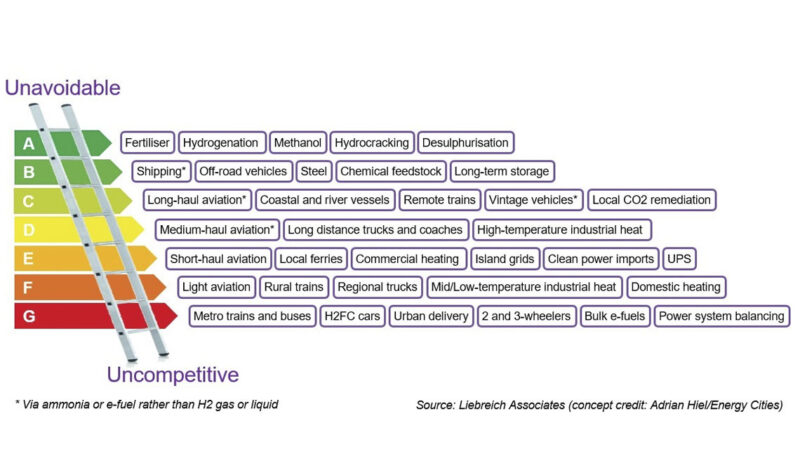
Is hydrogen really more environmentally friendly than fossil fuels?
It depends on how it is produced. Indeed, hydrogen only very sparsely exists in natural form in nature, and it therefore needs to be produced before it is used. Here is a good moment to discuss about colors of hydrogen. We regularly hear in the press about green hydrogen, blue hydrogen, grey hydrogen, etc. there is a whole rainbow of colors. But what does each of these colors mean? Let’s look at them individually:
- Green hydrogen: Hydrogen produced by the electrolysis of water, powered by renewable electricity. This is most often the color referred to when we discuss the hydrogen economy.
- Grey hydrogen: Hydrogen produced by the cracking of methane using steam. This process is the traditional process for producing hydrogen for refineries and ammonia production. The downside of it is that 9 tons of carbon dioxide are released for every ton of hydrogen produced, making this process the source of over 3% of the global carbon impact.
- Blue hydrogen: That is grey hydrogen with the difference that carbon emissions are captured and stored.
- Turquoise hydrogen: Hydrogen produced by methane pyrolysis.
There are many other colors, but these are the main references.
If using green hydrogen to replace fossil fuels, it almost systematically leads to reduced environmental impact, with a carbon impact generally between 1 and 4 tons CO2 per ton hydrogen.
As a general rule, it is recommended to talk about carbon footprint rather than of colors, because each color encompasses a large array of nuances, with a large array of carbon footprints.
Coming back to the question regarding whether hydrogen actually reduces the environmental impact, as a rule of thumb, grey hydrogen very rarely leads to a reduction of carbon footprint, green hydrogen almost always leads to reduction in carbon impacts, blue and turquoise are nuanced, it depends.
Another aspect to look at is of course the use-case. The main strength of hydrogen is its energy density. Therefore, in use-cases where energy density is key, the reduction of carbon impact will be more significant than when alternatives such as electricity can be used efficiently. When green hydrogen is used to replace existing grey hydrogen applications in the fertilizer or refinery sector, it also almost always leads to significant carbon footprint reductions.
Why are critics still pointing out the environmental impact of green hydrogen?
There are a few aspects to take into account when talking about green hydrogen. The first is to use hydrogen in use-cases where it makes sense thermodynamically and economically, not being carried away by the buzz around hydrogen.
Then, green hydrogen needs inputs of wind and solar based electricity. The environmental impact of the production of solar panels, wind turbines and electrolyzers needs to be taken into account in the carbon footprint. When these assets are installed in regions where the potential of wind and solar is low, the energy return of these assets will be low, increasing the carbon footprint of the final hydrogen produced. This is why producing green hydrogen in regions such Australia, Brazil, Middle East, etc. has a very high potential.
Finally, the carbon impact of hydrogen leaks need to be accounted for. As hydrogen is a very small and reactive molecule, it leaks very easily from storage and transportation vessels. When hydrogen leaks, it has a global warming potential of 11, meaning that every ton of hydrogen leaking has the same carbon footprint as 11 tons of CO2 in the atmosphere.
What’s the link between hydrogen and ammonia?
First, hydrogen is the most critical feedstock in the production of ammonia for fertilizer, having sawn a link between these 2 molecules for more than a century. The decarbonization of the ammonia value-chain, which is responsible for 1,5% of the global carbon footprint, is basically synonymous with the decarbonization of the hydrogen production step in the ammonia value-chain.
Then, ammonia can be used as a hydrogen carrier. As alluded previously, hydrogen is very hard to transport at scale, due to its gaseous form, its lightness and explosivity. Ammonia on the other hand is liquid at -33oC and has a very low risk of explosivity. Therefore, ammonia (with formula NH3), can be used to carry hydrogen (with formula H2), bound to a nitrogen atom. In the form of ammonia, hydrogen can be transported at scale intercontinentally. At the receiving end, it is possible to form hydrogen again in a process called ammonia cracking.
With this value-chain, it is possible to unlock intercontinental trade of green energy. Renewable energies would be produced in locations with a high renewable potential and converted to green hydrogen. Then, by combining hydrogen with nitrogen in the Haber-Bosch process, hydrogen in the form of ammonia can be shipped to locations with high energy demand and low renewable potential, such as Europe or East-Asia. There, ammonia can be recombined to hydrogen and used as clean energy in hard-to-abate sectors, such as heavy-mobility or heavy industries, following the “hydrogen ladder” model.
What is Proton Ventures’ role in the hydrogen economy?
As an engineering and project development company specialized on ammonia, Proton Ventures contributes as follows in the development of the hydrogen economy:
- Project Development: Proton Ventures develops large-scale green ammonia export hubs, together with its partners. One example is in the port of Pecem, Brazil, where Proton Ventures, together with the TransHydrogen Alliance, Casa dos Ventos and Comerc, is developing a 400kT/y green ammonia production facility for exports to Europe, with a planned upscale to 2,2MT/y.
- Engineering for ammonia production: Proton Ventures provides its engineering expertise to engineer and design ammonia production facilities based on green hydrogen.
- Engineering and EPC for ammonia storage: Proton Ventures is designing and building storage terminals that allow for the export and import of ammonia.
Cracking expertise: Proton Ventures has developed extensive engineering expertise for cracking, allowing us to select the best technologies and design cracking facilities to recombine ammonia into hydrogen at import locations.